Dr Ewan Goodier is Product & Business Development Manager at Eclipse Magnetics. He has worked extensively in magnetic system design and has a PhD in Electrical Machine Design and Permanent Magnets. Here, he answers some common magnetic questions (click on the question to see the answer).
Ask The Expert
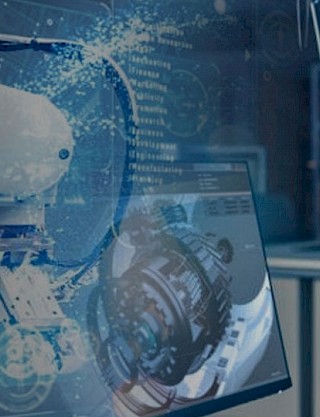
Consult the Magnet Doctor
Your Questions About Magnetics Answered Here By The Experts At Eclipse
What Are Magnets & What Is Magnetism?
A magnet is an object that can create an invisible magnetic field which can be used to interact with other magnetic fields (attraction or repulsion) or to attract to ferromagnetic materials such as iron, mild steel, cobalt and nickel. Magnetic fields always exit the magnet from its North pole and enter the magnet at its South pole.
There are three types of magnet. Permanent Magnets (Hard Magnets) produce a magnetic field all the time. Electromagnets produce magnetic fields only when electrical current passes through an electrical conductor (ideally in the shape of a coil). Soft magnets are temporary – they only produce magnetic fields while an external magnetic field is present and lose nearly all their magnetism once the external field is removed.
Magnetism is the physical event due to the forces occurring between magnets – it is noticed as attraction or repulsion. All materials will experience magnetism (except a vacuum). Some are strongly attracted to magnetic fields (ferromagnetic materials). Some are weakly attracted (paramagnetic materials). Some are actually very weakly repelled (diamagnetic materials). Some are attracted reasonably strongly (antiferromagnetic materials).
Magnetism can only be seen with the aid of other materials (e.g. iron filings, ferrofluid, compasses) – the materials align with the lines of magnetic flux (the field lines) to indicate how the magnetism moves around the magnet (they can impact on the actual field paths). Magnetic field lines are 3 dimensional – they can travel in all directions and interact with all materials (they can even pass through vacuums).
Magnets and magnetism is everywhere around us and we often use magnets daily without even knowing it. Planet Earth is a magnet (it has a magnetosphere giving us North and South magnetic poles) – we are continually in a very weak magnetic field all the time. Modern cars contain lots of magnets (e.g. seat belt sensors). Computer hard drives contain magnets, as do CD players, loudspeakers, microwave cookers, magnetic drill bits, fridge door seals, shower door seals, compasses and many electric motors and generators. Your fridge may have magnets holding pictures and notes in place. Your doorbell is an electromagnet. Door release mechanisms where you press a button to allow the door to be opened use electromagnets. All electric motors use an electromagnetic principle to work (they all have current carrying conductors which create a magnetic field which is used to turn the motor). Car remote central locking devices use solenoids (solenoids are electromagnets). Particle accelerators use electromagnets. Magnetism is literally everywhere in our lives but we often simply do not think about it to realise it.
Magnetism is used to convert mechanical energy to electrical energy (e.g. generators, microphones), mechanical energy to heat (e.g. eddy current heaters, induction furnaces), mechanical energy to mechanical energy (clamping magnets, magnetic separation, magnetic pump couplings) and other applications such as MRI, sensors triggering, etc.
What Permanent Magnetic Materials Are There?
There are several permanent magnetic materials:
- NdFeB - Neodymium magnets – most powerful magnet material available. A rare earth magnet.
- SmCo - Samarium Cobalt magnets– copes with extreme conditions the best. A rare earth magnet.
- Alnico - Alnico magnets– copes with the highest temperatures. Low coercivity can be useful but can also be problematic.
- Ferrite - Ferrite magnets– corrosion resistant, weaker material than the others above but often has by far the lowest cost.
- Compression Bonded magnets – isotropic magnet material capable of multiple pole shapes. Due to binder has lesser performance than the source magnetic material used to give it its magnetic performance.
- Injection Molded magnets – isotropic magnet material capable of complex multiple pole shapes. Has less performance than the Compression Bonded material.
- Flexible magnets – extruded or calendared ferrite plus binder, often used in magnetic signage applications in sheet form.
- FeCrCo magnets – malleable material often used in hysteresis brake applications or compass needles.
- Electro-permanent magnets - these are electromagnets that contain permanent magnets. They are on and clamp until you turn on the current to cancel out the magnetic field to release them. We also supply electromagnets.
How Do I Link A Magnet Polarity to Earth’s Magnetic Polarity?
The Magnetics Industry defines magnet polarity by the definitions accepted by scientists globally.
The North pole face of any permanent magnet will align to point to the geographic North pole of planet Earth.
By the rule of unlike poles attracting this makes the geographic North pole of planet Earth a magnetic South pole.
So planet Earth’s North magnetic pole is actually located at its South geographic pole. And planet Earth’s South magnetic pole is actually located at its North geographic pole.
The above is the correct definition.
Where + and – are used for polarities, the Magnetics Industry would ordinarily regard + as North and - as South but whether the seller of such marked magnets meant this would not be certain (because the Magnetics Industry do not use such symbols).
Can I have a magnet with just one pole?
No. Wherever there is a North Pole on a magnet, there must also always be a South pole on that same magnet.
Field strength of magnets – does shape matter?
Yes – shape definitely affects any magnet’s performance.
Imagine you have two N35 NdFeB magnets. One is 2mm diameter and 20mm long. The other is 20mm diameter and 2mm long. Both will work in different ways. The size, shape, environmental conditions and the total magnetic circuit all affect the magnetic performance. The grade and temperature also affects performance.
Stacking magnets can give increasing performance (up to an upper height limit beyond which extra height gives less and less increase in performance).
A magnet clamping directly to steel and a magnet clamping through a gap will have different size requirements (the latter would need a bigger pole face as a first hint to get performance).
So, if you are not sure what magnet would be best, just ask for our technical assistance. We can calculate magnetic outputs from any magnet and we also design magnets to give the best possible performance for a given application. We have 2D and 3D FEA software.
Magnet pull strengths, magnet field strengths
The size and shape of a magnet affects its magnetic field output and also its maximum possible pull force (holding force).
Temperature, environmental conditions, the material being attracted (size, quality, shape, permeability, etc) all impact on the magnet performance, as does the distance between the magnet and the material being attracted.
A magnet will clamp onto a mild steel sheet 1mm away much better than it will clamp onto the same sheet steel 50mm away (a bigger air gap reduces performance). A 100mm diameter NdFeB Neodymium disc magnet will clamp stronger onto a 50mm thick mild steel slab than it will onto a 0.1mm thick mild steel sheet (thin steel cannot carry all the magnetism and saturates). The application affects the performance.
Every magnet will have a pull rating value (often stated in kilograms but really it should be in Newtons because it is a force – 1kg=9.82N). The pull force test assumes that the magnet is operating at the highest Br for its grade and it is attracting onto perfect mild steel that is so thick it can handle all the magnetism. The values are therefore comparative and indicators of potential performance capability. You may never get to achieve the pull forces in your real world application.
In theory, if you have a magnet clamped onto mild steel and you pull it away with just above the rated pull force, the magnet will probably just be removed. The pull force test value is therefore a force guaranteed to pull the magnet directly away from the ferromagnetic material (the pull being perpendicular to the ferromagnetic face that the magnet is clamped onto).
The pull force can be converted to a clamping pressure if you calculate the magnet pole face cross sectional area (really the overlap cross sectional area). Divide the force in Newtons by the area in mm2 and the pressure is calculated (in Pascals or N/ mm2).
The pull force is given as a pull of the magnet perpendicular to the ferromagnetic surface. But the magnets can slide along the surface with a shear force. The shear force is affected by levels of friction. As a quick rough rule of thumb, a magnet is likely to start to slide over a ferromagnetic surface if the shear force applied is about 1/5th of the pull force (20%). For a pot magnet, the shear force is about 1/3rd of the pull force (33.3%). But a rubber coated magnet or a polyurethane coated magnet has extremely high friction levels so the shear force is about 2 times higher than the pull force. Because these are rough guideline values we recommend that you test them in your application. For note, one possible solution for where shear forces are not high but sliding is not wanted is where there is a magnet a magnet is sunk into one unit and another magnet or mild steel piece is standing out proud in other unit to create a “plug and socket” effect between the two units allowing clamping without sliding.
Magnet polarity marking – making identifying pole faces easier
Most permanent magnets do not have their polarity marked when they are produced. So it is not possible to tell, just from looking at a magnet, as to which ends are North and South – you would need an electronic polarity tester (you could use a compass as well).
Any magnet face could be given an identifying mark. Usually it is the North Pole which is marked (the South Pole can always be marked instead if needed). The identifying mark could be as simple as a permanent marker pen dot, line, letter N or other markation (an indelible ink is used). A more “technical” method may be to have a dimple pressed or machined into the pole face during production of the magnet. Ink stamps could also be used (with the possibility of using various colours – although the ink may possibly come off over time).
A mark could be done by wire spark erosion to identify the Direction of Magnetisation axis on a magnet (perhaps where it does not matter whether a North or South is used provided a pole can be quickly identified).
Laser etching can be performed to mark the magnets. One method is to laser etch deep into the magnet surface before any coating is applied to the magnet then coating over the etch to leave visible ridges / channels. Another method is to etching away the nickel layer of the NiCuNi coated magnet to leave a darker mark (removing plating may pose a longer term corrosion risk for magnets such as NdFeB).
In some systems, the magnets are packed in e.g. tubes or boxes and are magnetised in their package and the package is then labelled with the polarity. This is sometimes done for speed, ease and lower cost and is usually done to a customer’s own requirements.
Smaller magnets are harder to mark (very small magnets may not be possible to mark). The marking method requested will usually carry a fee for performing the marking.
Do you supply assemblies?
Yes. Assemblies are what we excel at and what we prefer to supply. We will supply magnets in low quantities and high quantities. We will supply magnetic assemblies in low quantities and high quantities. Sometimes tooling charges may apply (it depends on what is required).
Simply contact us with your assembly requirements plus any drawings and our production team will review it all so we can provide you with a quotation for supply. With production capability in China (we have a Joint Venture in China) and UK, we are extremely competitive. PPAP to e.g. Level 3 is no problem. We can also look to hold stock in UK for Just in Time supply of complete magnetic assemblies if this helps.
We, our Joint Venture in China and our approved subcontractors have between us ISO9001, ISO14001 and TS16949. The quality of our magnets is assured. If you need a Certificate of Conformity for our magnets – just ask.
Can a magnet be demagnetised by applying an external magnetic field?
When a permanent magnet is produced it is initially unmagnetised and it has to be magnetised to saturation to give it its maximum magnetic performance. This requires placing the magnet inside an electromagnet that applies a field to the magnet to drive magnetism into it until the magnet is fully saturated to Bs (so it cannot align any more of its magnetic domains because they are already fully aligned with the applied magnetic field).
So an external magnetic field can magnetise an unmagnetised magnet. If you reverse the external magnetic field so it now opposes the magnet’s Direction of Magnetisation, the magnet will be experiencing an applied field which could, if strong enough, start to demagnetise (weaken) the permanent magnet.
So how large an external magnetic field do you require to start to partially demagnetise a magnet and to even fully demagnetise a magnet? The answer depends on many variables, in particular the magnet shape, the total magnetic circuit, the temperature of the magnet and the BH curve of the magnet material being demagnetised. Every magnet in in a magnetic circuit (the magnetic field from the magnet interacting with the all the materials (air, mild steel, other magnets, electromagnets, etc) surrounding the magnet and this gives a Pci (Intrinsic Permeance Coefficient) which gives a working point on the Intrinsic Curve for the magnet material.
If the Intrinsic Permeance Coefficient for the NdFeB magnet is known then an Intrinsic Load Line can be drawn from the origin (B=0, H=0) and where it crosses the Intrinsic BH curve the intersection on the Intrinsic curve is called the Intrinsic working point. If the magnitude of the externally applied demagnetising field is known (of magnitude Ha), the Intrinsic Load Line can be translated along so the start of the Intrinsic Load Line has moved from H=0 to H=Ha. The slope of the Intrinsic Load Line is the same but the Intrinsic working point on the Intrinsic curve has moved (the working point will have moved along the Intrinsic BH curve as the Ha increased in value). The working point on the Intrinsic curve will move as the applied field Ha changed in magntitude. When the externally applied field Ha is removed, the Intrinsic working point ‘recoils’ back but at a slope equal to the Intrinsic curve slope where H=0 and B=Br. If the Intrinsic working point due to the applied field Ha has not entered the region of the ‘knee’ of the Intrinsic curve, the demagnetisation of the magnet is minimal (the recoil slope may follow the original BH curve back towards Br), possibly so little it cannot be measured (the recoil follows the original Intrinsic BH curve)
If the Intrinsic working point has gone into the region of the ‘knee’ as Ha has been applied, when Ha is removed the recoil does not follow the original BH curve shape but recoils back at a lower value – a new BH curve shape has been produced which has a lower new Br (this is the effect of the demagnetisation – a weakened magnet due to a lower Br). Applying an external field again up to the same magnitude of the original Ha has no further demagnetising effect. The magnet is said to be ‘conditioned’ for that level of applied field.
If an even stronger Ha demagnetising field is applied then further demagnetisation will occur.
If the Ha equals Hc (the Coercive force), the magnet will appear to have no magnetic output whilst the Ha field is being applied. Removing the Ha field will have caused potentially severe demagnetisation (leaving behind a severely weakened magnet).
If the Ha equals Hci (the Intrinsic Coercive force), the magnet will appear to have a reverse magnetic field output whilst the Ha field is being applied. Removing the Ha field will have caused total demagnetisation (the magnet will appear “dead” - it will show no net magnetic performance).
It should be noted that for SmCo, most Alnico and NdFeB as the magnets heat up, Br and Hci fall which make the magnets more prone to being demagnetised because the “knee” is being brought closer to the Intrinsic working point. Ferrite is an exception because the Hci increases as the magnet heats up (so cold is a problem for ferrite).
There are some applications where the magnet needs to be weakened to give a reduced required performance (e.g. alnico assemblies for mass spectrometers). The method of deliberately weakening the magnet for use is sometimes called ‘conditioning’ / ‘stabilising’ and the process of weakening is known as ‘knocking back’ and externally applied magnetic fields can give this effect.
Externally applied demagnetising fields can also be applied to mimic the effect of a demagnetising high temperature exposure. So instead of exposing the magnet to a high temperature (for ‘thermal stabilising’) if you know by how much the magnet weakens from exposure to that high temperature then you can work out what level of Ha needs to be applied to do the same task using an electromagnet.
Magnets must be held securely in place when inside electromagnets otherwise they can move or rotate around. For example if you were to place a fully magnetised magnet loose inside an electromagnet and try to apply 3-5 Tesla to demagnetise it, the magnet would either spin about rapidly and possibly break due to the domains ripping the magnet apart because it was not restrained or the magnet would fire out of the electromagnet literally like a bullet being fired. When any magnet interacts with an applied magnetic field the magnet will want to align with the strongest part of the applied field and will reposition itself into best attractive alignment or get itself out to a position of least repulsion.
And, for completeness, if you place a magnetised magnet inside a solenoid with an external magnetic field in the same direction as the field from the magnet, it will not weaken the magnet. Should the magnet have been weakened by heat or by another external magnetic field then some of the magnetic domains would start to realign due to the magnetising field being applied and the magnet would start to gain some of its original performance until the magnet saturates at Bs if the applied field is strong enough (it would be remagnetising the NdFeB magnet).
How can a magnet be designed to better resist an externally applied magnetic field?
The Intrinsic Permeance Coefficient (Pci) of a magnetic circuit (derived from the total magnetic circuit), the applied field Ha and the Intrinsic Coercivity Hci all determine how the magnet will demagnetise. To resist demagnetising the following can be done:
- • Chose a grade with a higher Hci (takes the “knee” away from the Intrinsic working point).
- • Change material to one with a higher Hci.
- • Lower the temperature (NdFeB, most Alnico and SmCo).
- • Increase the temperature (Ferrite).
- • Change the magnetic circuit to increase Pci (less air gaps, more magnet length, more steel, etc – improve the magnetic circuit).
Attraction or Repulsion – what is the rule?
For magnets attracting onto ferromagnetic surfaces such as mild steel, either a North pole face or a South pole face will attract to the magnet. Magnets on non-magnetic surfaces (e.g. plastic) generally do nothing. The only exception is some metallic electrically conducting surfaces such as Aluminium and copper and you move a magnet quickly over the surface of the Aluminium or copper – eddy currents are generated which oppose the moving magnetic field (explained by Lenz’s Law) giving a feel of magnetic drag/resistance slowing the magnet movement down.
For magnets near other magnets use this rule. Unlike poles attract. Like poles repel. So a North on one magnet will attract a South on another magnet. A North on one magnet will repel a North on another magnet. A South on one magnet will repel a South on another magnet (with equal force to the North on North if comparing the same magnets). Typically (as a very rough guide) repulsion is about half the force of attraction (it depends on the design but the repulsion force is always a lower force value than attraction force). Why? Because repulsion is a much worse magnetic circuit – magnetic lines of flux leave a North face and must travel around to the South face (each line must have a start and end) and the path will always aim for the shortest / easiest route (from a North to a South pole, to ferromagnetic material, etc); when in repulsion the flux lines pass through more air which has a higher magnetic resistance (reluctance) which makes the magnetic circuit far less efficient – air gaps are minimised in good magnetic circuits).
What is Lenz’s Law?
Faraday’s Law is used to describe the magnitude of an induced electric voltage (an emf – electromotive force) when a magnetic field passes through an electrical conductor such as a wound copper coil. Lenz’s law is used to describe the direction of the current that passes through that coil.
Lenz’s Law states that when a magnetic field changes in an electrically conductive wire, it produces an induced current in that electrically conductive wire with a direction such that the induced current creates a magnetic field which actually opposes the original change in magnetic field. Fleming's Right-Hand Rule (mainly used in Generator current prediction) can be used to show the direction of the current. The electrically conductive wire could be copper wire for example.
Lenz’s Law also demonstrates how eddy current brakes (hysteresis brakes) work. A magnetic field is moving through an electrically conductive metallic plate (aluminium, copper, some brasses). An eddy current is generated within the metallic plate which creates a magnetic field to oppose the moving magnetic field. The opposing fields slow the plate down. The eddy currents make the plate hot (current x current x resistance, I2R, becomes a heat loss).
What is Faraday’s Law?
Faraday’s Law is used to describe the magnitude of an induced electric voltage (an emf – electromotive force) when a magnetic field passes through an electrical conductor such as a wound copper coil. It is the basis of how all electrical generators and electric motors and machine work.
The induced (generated) voltage is proportional to the size of magnetic field in the coil, the number of turns in the coil, and the speed of change in field. The magnetic field must pass through the coil (cutting the coil) and change magnitude and or direction as it does so.
- • E=-N.A.(dB/dt)
- • E=Generated Voltage (back emf, induced voltage), sometimes shown as a V rather than an E (voltage)
- • N=Number of turns
- • A = coil cross sectional area
- • B=magnetic field in the coil
- • T=time
dB/dt = rate of change of magnetic field (as a magnetic field passes through a coil the coil starts to see a rising field which then peaks and then starts to fall, possibly with the field direction reversing depending on the design). So to create a higher generated voltage, you need more turns, or a higher magnetic field (e.g. stronger magnet, higher grade of magnet), a faster rate of change of field (e.g. faster rpm speed, better magnet design, more coils per revolution, more magnets per revolution, perhaps even more poles) or a combination of any of the above (we are assuming the coil area is fixed).
Lenz’s Law and Fleming’s Right Hand Rule help to give the induced current direction. Can this be used to predict how much power I can get? It depends on your design application and it is not straightforward. Motors and Generators take a lot of design work to get a very good product. The above is merely a simplified guide to give you the basic knowledge.
Can I tap a thread into a magnet (so I can screw directly to it)?
No – all the NdFeB, SmCo, Ferrite and Alnico magnets are all brittle and will not take tapped theads – the threads will break. Instead, use a metal such as mild steel, stainless steel, brass, etc to put a thread onto and assemble the magnet to that metal part. A good example would be pot magnets.
Can I weld near magnets?
Not always easily. Where an electrical spark is near a magnetic field, the magnetic field can cause the electricity flow to move so you might risk uneven welding or untidy welding. An electric current is a flow of electrons (charged particles) – when a charged particle crosses a magnetic field, it is deflected by the magnetic field (this is how an electric motor works). Processes such as TIG welding is therefore affected – shunting the magnetic field away from the welding area using ferromagnetic pieces (e.g. mild steel) may help (in a similar way to using a keeper plate on a horseshoe to keep the magnetic paths in a deliberate circuit). Laser welding should be fine, as should ultrasonic welding. Be mindful of keeping the magnets cool because if they get too hot they could permanently demagnetise (weaken).
Longevity of magnets – how long will a permanent magnet stay magnetic?
How long any magnet retains its magnetism for will depend upon the application and the environment that magnet is placed in.
If a permanent magnet is well looked after, not damaged, kept at room temperature, kept in a dry environment away from moisture, corrosion risks, radiation and external magnetic fields, it will in theory remain magnetic indefinitely (i.e. it could outlast the product lifecycle). As a rough guide based on well looked after magnets over 10 years the drop in performance for high permeance coefficient magnets should be very low e.g. nearing 0% for NdFeB, nearing 0% for SmCo, under 2% for ferrite and under 3% for alnico. In practice the conditions are not ideal so the long term performance could well be worse (or far worse) than stated (so please do not use the guideline values as to be expected values – you could be disappointed).
However, taking a magnet such as an NdFeB Neodymium magnet to an excessive temperature will start to demagnetise the NdFeB Neodymium magnet (irreversible but recoverable loss) weakening it and extreme heat will completely demagnetise it. Exposure to higher moisture environments will expose the NdFeB Neodymium magnet to a corrosion risk (especially salt spray and marine environments). Heat can also play a part in the corrosion process. Corrosion causes a permanent loss in magnetic performance and can eventually cause a total magnetic failure. It is for this reason that, in safety critical applications where moisture and corrosion is possible, SmCo Samarium Cobalt magnets or Ferrite magnets are preferred as these will not corrode when in damp or wet conditions (and why SmCo Samarium magnets are often a first choice in military and aerospace applications). How long it takes for corrosion to be seen really depends on the application and the environment. In dry conditions it may never be seen. In damp conditions it could take years, months, perhaps in damper conditions weeks. In wet conditions, corrosion could be noted in weeks or even days. If the coating is damaged, corrosion can occur faster. The coating type will help in minimising corrosion risk e.g. zinc plated NdFeB Neodymium magnets with a rubber overcoat layer can perform better than a standard Ni-Cu-Ni layer and Everlube coatings are reported to be good for corrosion resistance. The latest grades of corrosion resisting NdFeB Neodymium magnets have also improved the alloy’s resistance to corrosion (although still not preventing it, the time taken before corrosion is seen is extended – these grades do carry a price premium).
SmCo Samarium magnets do not corrode as badly as NdFeB Neodymium magnets. Alnico magnetsdo not corrode badly either. Both are much better with water than NdFeB (both should be given some protection against corrosion but their rates of corrosion are not as bad as NdFeB). Ferrite will not corrode with water.
If the NdFeB is taken to too high a temperature it will start to magnetically weaken (by definition, this is an irreversible but recoverable loss – only recoverable by remagnetising). Too high a temperature and the magnet can be totally demagnetised and extreme heat can change the alloy composition resulting in an irreversible irrecoverable loss (in that remagnetising will not regain the original performance). The same applies for SmCo, Ferrite and Alnico. Alnico copes with the highest temperatures followed by SmCo, Ferrite then NdFeB. Ferrite will demagnetise when it gets too cold (because ferrite, unlike the others, gets a significant reduction in Hci as it cools down – SmCo and NdFeB increase Hci as they cool down).
All magnets are brittle – striking the magnet will potentially damage it, perhaps with sections breaking away. For note, it is possible to get sparks when SmCo and NdFeB chips off, so magnet use in explosive environments is not recommended unless it is within a protected housing assembly (e.g. plastic encapsulated). A harder strike on any magnet will break the magnet into pieces – the shards can be very sharp and the resulting broken magnet will usually collect to itself with shards rotating around to leave a weakened unusable ‘scrap’ magnet.
It is therefore possible to have a permanent magnet that will stay magnetic forever but your application may not make this possible. Your application, its environment and the magnet choice and magnet design will all affect how well magnets last and which magnets should be used (or avoided). If a guaranteed long lifespan is required, SmCo or Ferrite may possibly be better suited magnetic materials. If in any doubt please contact us for advice.
Will a magnet affect my credit cards and other magnetic stripe cards?
Magnetic stripe cards are also known as mag stripe cards or swipe cards. They are a popular choice for cards that store data e.g. bank cards; ATM cards, gift cards, membership cards, phone cards, hotel key cards; etc. There are two types of material generally used with magnetic strips on Credit Cards and other magnetic cards – LoCo and HiCo. LoCo has lower coercivity and is easier to be demagnetised (“wiped”). HiCo has higher coercivity and is more resistant to being demagnetised (“wiped”) but they can be demagnetised with stronger (more powerful) magnetic outputs (such as NdFeB magnets).
HiCo magnetic stripe cards have a coercivity as high as 4000 Oersteds; however 2750 Oersteds is common for many HiCo cards. Most credit cards have at least 2,750 Oersteds.
LoCo magnetic stripe cards have a coercivity typically around 300 Oersteds. ; is best suited for cards used infrequently and/or where data is routinely changed, such as with hotel key cards. HiCo cards are best for cards used frequently and that are expected to have a long life. Most credit cards use at least 2,750 Oe and are considered HiCo.
A HiCo magnetic stripe cards typically takes 0.4T (4000 Gauss) to demagnetise the stripe but even a field as low as 1250 Gauss could demagnetise sections of the stripe. A LoCo magnetic stripe cards typically takes 0.03T (300 Gauss) to demagnetise the stripe but even a field as low as 100 Gauss could demagnetise sections of the stripe. A rough guide is to keep the field below one tenth of the coercivity to have the best chances of avoiding demagnetisation e.g. less than 0.04T (400 Gauss) for HiCo and less than 0.003T (30 Gauss) for LoCo.
Magnets with Pacemakers and Heart Defibrillators
We do not recommend that people with pacemakers, heart defibrillators and similar devices use magnets. We recommend that they seek guidance from their own doctor in relation to magnets near their device.
The World Health Organisation (WHO) Fact Sheet 299 and the International Commission on Non-Ionizing Radiation Protection (ICNIRP) both offer information on magnetic field exposure levels which are useful independent sources of information.
The WHO Fact Sheet 299 gives the following information:- “Static magnetic fields affect implanted metallic devices such as pacemakers present inside the body, and this could have direct adverse health consequences. It is suggested that wearers of cardiac pacemakers, ferromagnetic implants and implanted electronic devices should avoid locations where the field exceeds 0.5 mT. Also, care should be taken to prevent hazards from metal objects being suddenly attracted to magnets in field exceeds 3 mT.”
Magnetic fields if strong enough can interrupt the normal function of devices such as defibrillators, pacemakers and similar devices. It is for this reason that we suggest that people with such devices should never use magnets unless given specific special instructions by their doctor. Magnet companies are not medical experts.
Will a magnet affect my watch?
Magnets can affect the performance of mechanical watches. They can magnetise sections within the watch such as the spring mechanisms which could make the watches operate faster or slower.
We recommend that you remove your watch if you are going to be working with magnetic fields to prevent any risk of damage to the watch. If a watch has been magnetised by accident and no longer works as required then some specialist watchmakers may be able to demagnetise the watch to get it working again.
There are some watch manufacturers who offer non-magnetic watches to ISO 764 which should mean that the watches may be able to resist magnetic fields up to 60 Gauss in magnitude without damage but with the watch losing or gaining up to 30 seconds in each day. A few high specification watches claim to withstand fields up to 1000 Gauss (0.1T). We still recommend that you keep mechanical watches away from magnetic fields if at all possible.
But also note that analogue quartz watches can have their motors sped up or slowed down by magnetic fields but as soon as the field is removed the motor should continue to operate normally again.
Will a magnet affect my cochlear implant (Hearing Aids)?
Strong magnetic fields may affect the performance of the speakers. Above 0.2T permanent damage may be possible. Between 0.02T and 0.2T the damage is more a temporary interference.
Will a magnet affect my USB data stick or compact flash memory cards?
USB sticks and compact flash memory cards do not contain any magnetic data (they are non-magnetic storage media). Therefore they should not be damaged by magnetic fields.
Will a magnet affect my mobile phones or camera?
Mobile phones and cameras usually do not contain magnetic storage media. They usually contain non-magnetic storage media. As such magnetic fields near such devices should not be able to delete any data.
The only possible effect is that some devices have built-in compasses (magnetometers inside them) and the magnetic fields would affect their functionality. If you have such devices you may wish to keep strong magnetic fields away from them to protect them but we have no information in relation to any possible damaging effects on the magnetometers.
But there are covers with magnetic closures readily available on the market, which suggest that smaller less powerful magnets are generally safe with such devices. However very strong magnetic fields might be able to damage parts such as built-in speakers.
So, if you are in any doubt, it would be best to consider keeping such devices away from strong magnets.
Will a magnet affect my hard drive?
Hard drives contain magnets. But very strong magnetic fields can partially or fully erase the data on them.
We recommend keeping magnets away from such devices – as a rough guide at least 300mm away (further away if the fields are very strong).
Some people use magnets to deliberately delete the data on hard drives. Using magnets does not guarantee complete data erasing (“wiping”). Specialist data removal software may be a better option to consider as well.
What happens if radiation is applied to magnets?
Rare Earth magnets can be demagnetised by radiation. The NdFeB Neodymium Rare Earth magnets do not perform as well as SmCo Samarium Rare Earth magnets when it comes to radiation effects. E.W. Blackmore, (TRIUMF, 1985) and A.F. Zeller & J.A. Nolen (National Superconducting Cyclotron Laboratory, 09/87) demonstrated SmCo having a better performance, with Sm2Co17 offering 2-40 times better radiation resistance than NdFeB. Some NdFeB grade are demagnetised to half their maximum performance with a proton beam radiation of 4 x 106 rads and are completely demagnetised with a proton beam radiation of 7 x 107 rads. A rule of thumb is to select magnets with higher Hci values, designed to operate at high Pci and, where possible, to have radiation shielding protecting them when being subjected to any levels of radiation. The user of the magnets would need to test for effectiveness of the magnets as the magnet suppliers do not have the equipment to test for suitability of magnet grades for environments with raised levels of radiation.
Are Rare Earth magnets actually rare?
No – the NdFeB Neodymium magnets and SmCo Samarium magnets (both Rare Earth magnets) are readily available. There is nothing rare (or scarce) about them.
They are called Rare Earth magnets because the Neodymium and the Samarium are classified as Rare Earth elements (the term ‘Rare Earth’ comes from the old and lengthy timescale methods of extracting the elements from the ores from the ground – modern methods are far more effective, quicker and efficient). These elements are actually quite common and NdFeB Neodymium magnets are made in hundreds of tonnes per year. They are not scarce. For example there is more Neodymium element (Nd) than there is Gold (Au).
The average concentration of Rare Earth elements in the Earth's crust ranges from around 150 to 220 ppm (parts per million). Putting into context zinc is 70 ppm and copper is 55ppm.
The Rare Earth elements (sometimes called REEs) are in the lanthanide series of the periodic table, ranging from lanthanum (atomic number 57) to lutetium (atomic number 71). The Rare Earth elements have to be mined from the ground. Most mine deposits contain a large variety of elements, some being radioactive (uranium, thorium and radium – making safe mining expensive and complicated).
Light rare earths (LREs - lanthanum, cerium, praseodymium, neodymium, promethium, samarium and europium) are generally much more common than Heavy rare earths (HREs- gadolinium, terbium, dysprosium, holmium, erbium, thulium, ytterbium and lutetium). Each mine has its different mix of elements in varying concentrations.
The Rare Earth elements often occur in multiple mineral types which complicates the separation process resulting in each mine having its own variation of separation methods because each mine has its own mixture of ores, minerals, elements and concentrations of each.
And to make the rare phrase less important, just one mine in China (Bayan Obo) produced 55k tonnes in 2009, which over 45k tonnes coming from a range of other mines in China in the same year. Mines in USA, Brazil, Russia, India, and Malaysia also exist and are being further developed with new mines being developed across the glove.
And vast deposits have been identified in the Pacific Ocean floor near Japan with exploratory work being carried out (claims of one square kilometer having enough rare earth concentrations to possibly supply up to a year’s worth of global demand). So the longer term supply of Rare Earth elements appears to not be an issue.
NdFeB magnets, Neodymium Iron Boron magnets, Neo magnets, Neodymium magnets, NIB magnets – are they the same thing?
Yes. The full name for the NdFeB magnet is Neodymium Iron Boron. The other names are used to describe the same magnet. Just be careful if a magnet is only called “Rare Earth” magnet – although NdFeB is a rare earth magnet, SmCo is also a rare earth magnet. SmCo and NdFeB are different in performance.
What is a BH curve? What is Br? What is Hc? What is Hci? What is BHmax?
A BH curve characterises the potential performance of a permanent magnet material. For a permanent magnet, the BH curve is the actually the second quadrant demagnetising curve (it could be the . The BH curve varies with temperature. The BH curve also changes with each grade. The BH curve is often empirical or based on empirical data. A permeameter is used to test each magnet sample to create the BH curve. Each BH curve should show three important values, Br, Hc and Hci. The Br is the remanent induction and is the value of the Normal and Intrinsic curve where the applied field H=0 – the higher this value the higher will be the potential magnetic output from the magnet. The Hc is the coercive force and is the position on the Normal curve where B=0 – the higher this value the higher an applied external demagnetising magnetic field must be before the magnet shows no net magnetic output (it gives an idea of possible resistance to demagnetising but Hci is a better value to use). The Hci is the intrinsic coercive force and is the position on the Intrinsic curve where B=0 – the higher this value, the higher an applied external demagnetising magnetic field must be before the magnet is completely demagnetised (so it gives a true idea of how well the magnet will cope with demagnetising forces). On the BH curve the normal curve has a value for B and a value for H – at each point on the normal BH curve the mathematical value of B times H is a BH energy product and at some position this BH value is a maximum called BHmax. The BHmax value also indicates potential performance – if you design a magnet to work at its BHmax position the magnet has minimum volume for maximum performance but in some designs, especially Alnico designs, the magnet could be more prone to demagnetising (so BHmax is better thought of as a performance indicator).
Magnetic designs using FEA, BEA or hybrid solvers require BH curve data.
What should I consider relating to the safety of magnet usage?
Take great care when using magnets. Be safe. Do not rush. Protect yourself and everyone who may be near the magnets. Perform a Health and Safety risk assessment before handling or using the magnets for everyone who may be near the magnets. Wear appropriate safety equipment (e.g. safety goggles).
Magnets are often more powerful than people think and many people are surprised at how strong some of them can be. Never be complacent. Always assume magnets are extremely powerful to start with and you will increase your chances of keeping safe.
Any person wearing pacemakers, defibrillators or any other similar equipment should never handle, wear or touch magnetic materials and should seek medical advice from their doctor on being at or near magnetic fields.
Some magnets can be very powerful and could be very dangerous if mishandled or handled in an unsafe manner. All persons should be given suitable Health and Safety training before considering handling magnets (to be safe), carrying out a Health & Safety risk assessment as necessary. Magnets can clamp to other magnets and to ferrous objects with high forces possibly being involved – finger pinching or limb trapping may be possible for the more powerful magnets.
Always take appropriate care. Wear appropriate protective equipment e.g. safety glasses. If an accident were to happen such as tapped hand that cannot be released you may need to go straight to your nearest accident and emergency hospital for immediate medical assistance.
We recommend that children should not handle magnets unless they are known to be very weak and suitable safe enough for use (e.g. EN71 approved magnetic toys and games, perhaps fridge magnets, perhaps flexible rubber magnets).
Never allow children to use magnets on their own – a suitable trained competent adult must supervise at all times. If in doubt, keep magnets away from children.
Keep magnets away from credit cards – they could wipe the data on the magnetic strips.
Keep magnets away from sensitive electronic equipment such as computer hard drives, meters, etc.
Magnets are brittle and can break, ship or shatter with sharp shards if they hit another magnet or other components too fast (more of an issue with the more powerful magnets or very fast collisions). Appropriate safe handling could avoid this but we recommend protective equipment such as safety goggles should be worn.
Never eat or ingest magnets – if you do, go straight to your nearest accident and emergency hospital for immediate medical assistance.
Can we produce custom shape magnets for you?
Yes – provided the manufacturing methods allow for the shape to be produced. We specialise in custom magnet and custom magnetic assembly production. Please let us know exactly what you want and we will look at it to provide a quotation. There may be minimum order charges and, depending on what is required, possibly also tooling charges. If there’s a problem with the request we will contact you to let you know the reasons so we can look to agree on a more feasible alternative.
Can we produce the stock magnet size but in a different grade?
Yes – provided the grade exists and it is feasible (e.g. N52 grade magnets require a higher press tonnage limiting the maximum size more than the lower grades of NdFeB such as N30). There may be minimum order charges if we can produce the magnet.
Can I get a quotation for much higher quantities?
Yes. If you are looking for a few packs then the chances are that the pack prices would still apply. But if you are wanting many multiples of packs (hundreds, thousands, tens of thousands, millions or individual magnets) then a quotation from us would be more appropriate for you needs. Just for reference, our lead times are based on current production lead times at the time of quoting and we always try our best to meet or beat our lead times so you are not disappointed.
Can I buy magnets and magnetic assemblies in bulk?
Yes. Please contact us with your requirements and we will provide you with a quotation.
I have an idea for a magnet design but I don’t know if it is possible – can you help?
Yes – we specialise in custom magnet production and custom magnetic assembly production and are happy to assist. If the magnet is possible, we will tell you, if it could be improved we would give you the suggestions, if it is not possible we would tell you why and, if alternatives are possible we would discuss them with you.
I cannot find the information I am looking for – what can I do?
We have tried to make our web sites informative and comprehensive and we will continually look to improve and amend them. But if you have not found the information you were looking for please contact us and we will help you as best we can. We would also look to add any new information which will further enhance the knowledge of our customers. Good use of information creates knowledge and good use of knowledge creates wisdom. If we can improve our sites for the benefit of our customers we will look to do so as soon as we can.